Physicists at the Princeton Plasma Physics Laboratory, General Atomics and other research institutions have made new observations that help to understand one of the major barriers to developing fusion energy.
The new findings, published in two back-to-back articles in “Physical Review Letters” on March 12, present novel observations from experiments that sought to control damaging heat bursts that routinely occur in a type of fusion machine called a tokamak. The work builds on previous observations made at the same tokamak, located in San Diego, Calif., and known as DIII-D.
Although fusion power is still in the experimental stage, the findings represent a step forward in understanding how fusion reactors might one day feasibly provide an alternative to the combustion of fossil fuels.
“These two papers actually contribute significantly to our understanding of the physical processes,” Alberto Loarte, a physicist and section leader at ITER — the world’s largest experimental tokamak reactor —who was not involved in the study, said. ITER will benefit from the team’s measurements, he added.
Inside a tokamak, a plasma — made up of charged ions and electrons — is confined in a donut-shaped chamber by magnetic fields. A successful, energy-producing fusion reactor would have to harness the energy created from matter when ions in this plasma collide and fuse with each other. This energy release is described by Albert Einstein’s observation that energy equals mass times the square of the speed of light.
However, maintaining the plasma at its hot, high-energy state presents a number of challenges. One challenge is the control of heat bursts caused by instabilities called “edge-localized modes.” Appearing like solar flares escaping the surface of the sun, which is a large ball of plasma, these ELMs allow a large amount of heat to escape all at once, rather than in a lower-energy, steady stream.
ELMs create difficulties for physicists hoping to create a fusion reactor, Loarte said.
“If there are many of them, they can erode the wall of the reactor very quickly,” he said. “This is a problem because we would have to stop the operation to replace the components.”
Previous work at DIII-D revealed that applying small, additional magnetic fields to the plasma helped to suppress ELMs by allowing heat to escape more gradually, Mickey Wade, program director at DIII-D, said. Theoretical work at other fusion research centers has since made several predictions about how this suppression might occur, and focus has been directed at tiny ruffles that appear at the edge of the plasma, allowing heat to leak gradually instead of building up into bursts.
However, experimental observations to support this theoretical understanding were lacking, he said.
“Until these articles were published, there wasn’t a clear scientific understanding of the underlying mechanisms that led to the elimination of the ELMs,” he explained.
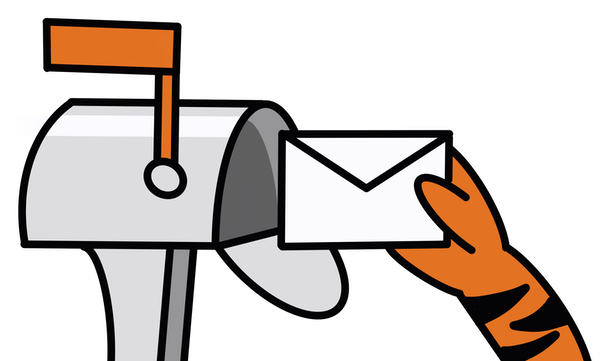
Something of a crucial step was the team’s decision to change the placement of sensors measuring the plasma’s behavior inside the tokamak, said Raffi Nazikian,the second paper’s lead author and a research physicist at PPPL.
The second paper is entitled,"Pedestal bifurcation and resonant field penetration at the threshold of edge-localized mode suppression in the DIII-D tokamak."
Previous experiments had placed sensors only on the longer, outer edge of the donut ring. However, the scientists in these experiments placed sensors along the shorter, inner edge of the donut ring, Nazikian said.
“That’s usually a place that is quite inaccessible and rather hostile to measurement,” he explained. “But it was on the inside of the donut that all the interesting action was taking place.”
Combined with high resolution measurements and finely-tuned application of magnetic fields to the plasma, this new position allowed the team to see things they hadn’t seen before, said Carlos Paz-Soldan, lead author on the first paper anda scientist at General Atomics.
His paper is entitled"Observation of a multimode plasma response and its relationship to density pumpout and edge-localized mode suppression."
“We found that we could excite a different ruffling of the plasma, one that was concentrated on the inside where the new sensors were located,” he said.
While plasmas exhibiting ELMs often showed ruffles on the outside edge, the plasma that had reduced ELMs had lots of little ruffles along the inside edge, extending up to the top and bottom surfaces of the donut, Paz-Soldan explained.
These smaller, inside-edge ruffles were associated with gradual heat release from the plasma, Nazikian said.
These new observations have already supported calculations made by physicists at other facilities, predicting that secondary ruffles could occur in some conditions, Wade said. The team cannot completely confirm that these ruffles reliably cause the suppression of ELMs, not least because the effect was only observed in a small fraction of the tokamak.
“The real question is, as we expand and go to different operating space, does this correlation between the second ruffle and the ELM suppression still exist?” he said.
It will also be important to scale the experiment up for different tokamaks, Ken McClements, a theoretical physicist at the Culham Centre for Fusion Energy who was not involved in the study, said.
“One of the questions that’s raised is ‘Can similar effects be seen on other machines?’ ” he said. “It’s always good to try to see how generic this behavior is, or whether it’s peculiar to one particular tokamak.”
Another concern is the production of instabilities in the plasma by the very act of trying to suppress ELMs. One of the next directions for research will be to find out how to apply magnetic fields to retain the benefits of ELM suppression without introducing other problems into the tokamak, Nazikian said, adding that theoretical work will be crucial to develop this understanding.
With these trade-offs in mind, other facilities such as ITER will be taking advantage of the new results to see if they can optimize ELM suppression in their machines, Loarte said. Ultimately, the need to make fusion in tokamaks a financially viable method of energy production puts constraints on how to design future reactors, he explained.
“You can always limit the erosion of the walls of the reactor at the cost of not producing any energy,” Loarte said. “So we need to optimize the erosion compared to the energy production, because all of these integration issues come at a price.”